Cite as: Benner, S. A. (2022) “Science, Hype, and Prebiotic Chemistry”. Primordial Scoop, e20220618. https://doi.org/10.52400/OQOB2950
From time to time, these pages discuss ways in which different scientific cultures approach the same problem, often with different demands.
For example, a 2010 paper reported that phosphate could be replaced by arsenate in the DNA of some bacteria [1]. This report was not seen to be “exceptional” by physicists [2] and geologists. Indeed, geologists see phosphate replaced by arsenate in rocks all of the time, although most geologists understand the difference between minerals and biomolecules.
However, chemists and biologists saw the proposed replacement as very exceptional. Including me.
Why? In my training in the culture of chemistry, I was taught why arsenate is poisonous. If one understands the chemistry of how arsenate kills people, one understands why arsenate cannot replace phosphate to link together nucleotides in DNA.
The understanding requires a logical network with many “if-then” connections based on chemical reactions where arsenate and phosphate participate. At its end, the network hands the burden of proof to the “pro-arsenate team”, rather than the “con-arsenate team”. If the back-and-forth of the dialectic ends there, the proposition is denied.
Later, a physicist and a biologist collaborated with me to explore why these four different scientific cultures saw the same data very differently [7]. Remember, “culture” is what you think when you are not thinking.
Organic chemistry begins with “molecular structures”, models for how carbon, oxygen, nitrogen, hydrogen, phosphorus (or arsenic), and other atoms are linked together in organic molecules. Such models cannot be assembled by direct observation of the molecules; they are far (far) too small to see. Rather, they deduce the model by a network of logical steps.
The question posed by the 2010 paper on arsenic was fundamentally a “structure proof” question. Did a DNA analog in a specific bacterium contain oxygen-arsenic-oxygen atomic linkages? Or did it not?
Structure proof in chemistry is not “proof” in a mathematical sense. Rather, it involves many of these networks of “if-then” connections based on compounds and reactions that make and break atom-atom bonds. Compounds having unknown structures are experimentally converted by these reactions into compounds whose structures have previously been assigned. This creates a connection in the network, which allows us to make inferences about bonds in compounds whose structures are unknown.
In making such inferences, we must also make assumptions about how atoms move during the converting reactions. These assumptions are supported by other transformations with a similar reaction involving still more different compounds.
Sometimes, those inferences are wrong. A chemical conversion can rearrange atoms in ways that we do not expected based on analogous reactions in other molecular systems. This leads to a mistaken structural model for a molecule.
We teach examples of these mistakes to students of chemistry, so that they appreciate the challenge. Famous molecules like penicillin and strychnine were mistakenly assigned an incorrect structure at first. These mistakes were eventually corrected. When they were, another few links were added to the network, making it increasingly robust.
How does this all relate to prebiotic chemistry?
Last month, we published a paper reporting that ribonucleoside triphosphates interacting with basaltic glass gave polyribonucleic acid 100-200 nucleotide units long. Interestingly, and important to the significance of this result to the origin of life, andesitic glass did not work as well. Simple quartz powder did not work at all. I discussed this last week.
I found the simplicity of this process surprising, even disquieting. Yes, getting ribonucleoside phosphates on a Hadean Earth was itself a problem, discussed elsewhere on this blog. Our “discontinuous synthesis model” (DSM) for prebiotic chemistry focuses on making these triphosphates, without human intervention, in specific geological environments.
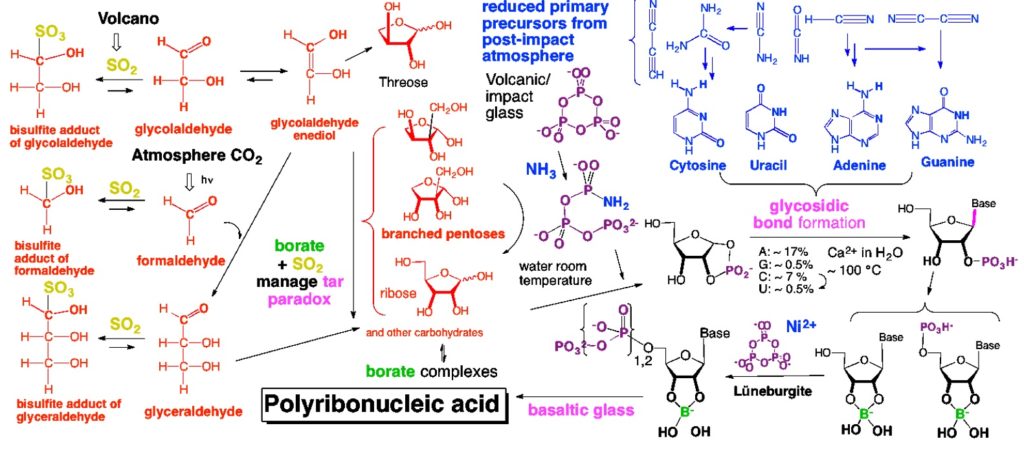
But I had expected that the last step, getting long RNA molecules from those triphosphates, would be much more difficult. And yet the process was simple on basaltic glass, which was everywhere on the surface of the Hadean Earth 4.35 billion years ago.
Of course, simplicity is a requirement for any chemical process to be relevant to the origin of life. But nevertheless, this level of simplicity seemed … well … ridiculous. At least to me.
In science, one has fun criticizing one’s own models and theories. Self-criticism is also a good way to train students in the intellectual discipline needed to practice science. And, as I have noted elsewhere, the DSM for prebiotic RNA formation can be criticized in many of its aspects.
For example, the DSM offers no idea of how homochirality emerged. We must worry about leakage of material from borate-managed carbohydrate maturation within the DSM, the part in red in the picture above. We must get the entire DSM process to work continuously. From this continuous process, we must calculate a “yield”, something very important in the culture of chemistry. We must distribute kits to high-schoolers, so that they can themselves observe this process.
Nevertheless, our report was picked up by Robert Service, a news writer at Science. He quoted several individuals not involved in the study. “This seems to be a wonderful story” whose “beauty is its simplicity”. Very nice.
Then Bob wrote that Jack Szostak, an expert in the field, disagreed, wanting more structure proof for the polyribonucleic acid made on basaltic glass. Bob quoted Jack as saying: “I find it very frustrating that the authors have made an interesting initial finding but then decided to go with the hype rather than the science” [emphasis added].
Those who have remarked that Jack was overly harsh by using the word “hype” should not be concerned. I have known Jack for 40 years, long before Jack Szostak was “Jack Szostak.” Jack is one of two people on this planet who appear committed to preventing my getting a swell head.
My wife is the second.
But this does offer an opportunity to discuss how classical structure proof works in chemistry. We start with how the structure of natural RNA was proven, classically.
Natural RNA has an easy reaction to convert its (then) unknown structure to (then) known structures, its nucleotide components. A phosphorus atom links the oxygen of a preceding nucleotide to the 5′-oxygen of the following nucleotide, a O-P-O arrangement of atoms. Because that phosphorus atom sits next to a hydroxyl group of the preceding nucleotide, treatment under alkaline conditions causes polyribonucleotide chains to fragment. A picture is worth 1000 words.
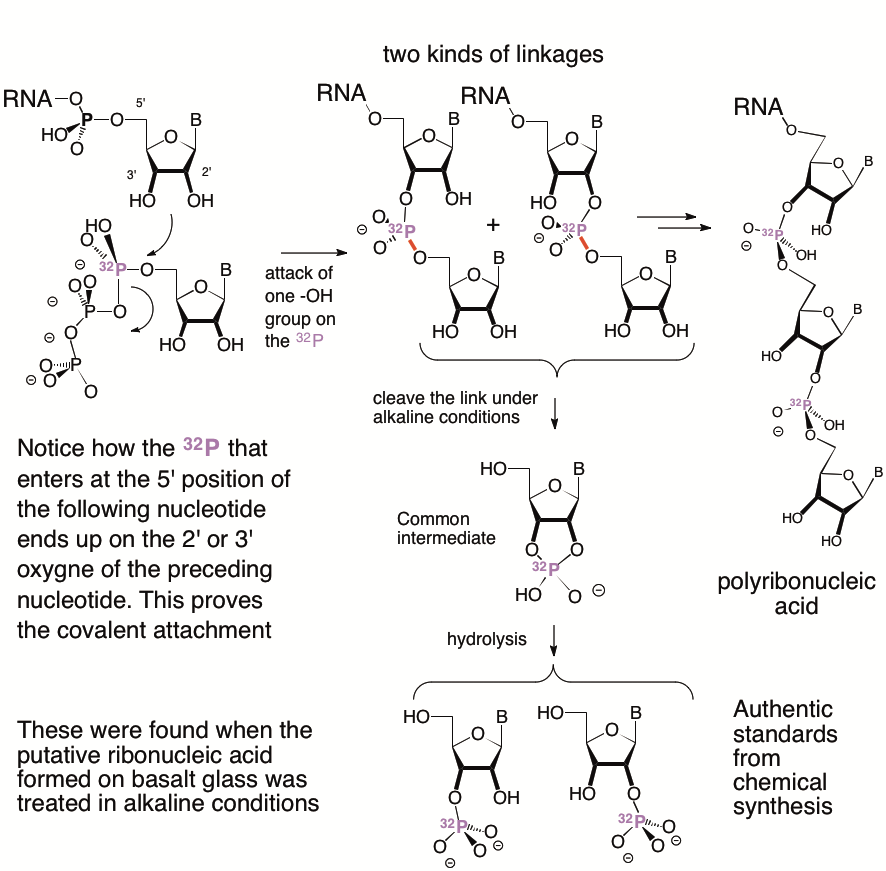
The immediate products in the fragmentation have new bonds. In particular, the fragmentation product each have a phosphorus atom bonded at the same time to both its 2′- oxygen and its 3′-oxygen of the preceding ribonucleoside. This is a “2′,3′-cyclic nucleoside monophosphate”.
The same cyclic product is formed regardless of whether the O-P-O bond links the 3′-oxygen of the preceding ribonucleoside to the 5′-oxygen of the following ribonucleoside, or links the 2′-oxygen of the preceding ribonucleoside to the 5′-oxygen of the following ribonucleoside.
Now, the 2′,3′-cyclic nucleoside monophosphate suffers a further reaction in the alkali where it is formed. It opens to give a mixture of nucleoside 2′-monophosphates and nucleoside 3′-monophosphates.
Thus, observation of abundant nucleoside 2′-monophosphates and nucleoside 3′-monophosphates in the degradation of polyribonucleic acid made on basalt would prove the existence of abundant O-P-O covalent links. Further, as shown in the picture, if the polyribonucleic acid came by polymerization of nucleoside 5′-triphosphates that had a radioactive phosphorus-32 attached to their 5′-position, the magenta 32P phosphorus that originated in the following nucleotide ends up bonded to the 2′- or 3′-oxygens of the preceding nucleotide.
Elisa Biondi, who led this project, did this exact experiment. She recovered 32P labeled ribonucleoside 2′- and 3′-monophosphates from polyribonucleic acid made by basalt from ribonucleoside 5′-triphosphates. These recovered products could not possibly have arisen unless a 2’à5′ or 3’à5′ O-P-O covalent linkage stood between them and the starting 5′-triphosphates.
A deduction from premises.
This is why the most important evidence proving structure in the Astrobiology paper relied on its detecting nucleoside 2′- and 3′-monophosphates in products created on basaltic glass.
Note that recovery of the known products does not let the chemist know whether the predecessor O-P-O linkages were 3′ -> 5′ or 2′ -> 5′. The same cyclic intermediate is formed from both. Information about the precise upstream O-P-O linkage is lost by the time one around to isolating the final products.
Of course, this was also true in the classical structure proof of natural ribonucleic acid. Thus, the term “polyribonucleic acid” in 1952, just 70 years ago, did not mean anything more than a polymer built from nucleoside units joined via phosphodiester O-P-O links.
It was not until 1953 that Dan Brown figured out that bionatural RNA on Earth was primarily 3′ -> 5′ linked. The year is significant; it is the same year that Watson and Crick published their structure for the double helix of DNA. That is, at the time that Watson and Crick were working out the folded structure of natural DNA, we still did not know the covalent structure of the links in natural RNA.
No matter what the details of the links, basalt glass makes polyribonucleic acid with covalently linked nucleotides. Further, since Elisa and Hyo-Joong Kim recovered lots of ribonucleoside 2′- and 3′-monophosphates, the polyribonucleic acid must contain many covalently linked nucleotides, joined by either 2′ -> 5′ or 3′ -> 5′ O-P-O arrangements of atoms.
A classical structure proof. Known degradation products obtained from an unknown precursor from reactions whose details we have understood for a century.
In other words: Basaltic glass makes polyribonucleic acid, defined as natural polyribonucleic acid was defined before Dan Brown’s 1953 work.
But that was not Jack’s concern, as we understand it. And it had nothing to do with “hype”.
Jack was evidently concerned that some nucleotides in the polyribonucleic acid might have both their 2′-oxygens and their 3′-oxygens linked to a following nucleotide. This would give a branched polyribonucleic acid. Another picture is worth 1000 words.
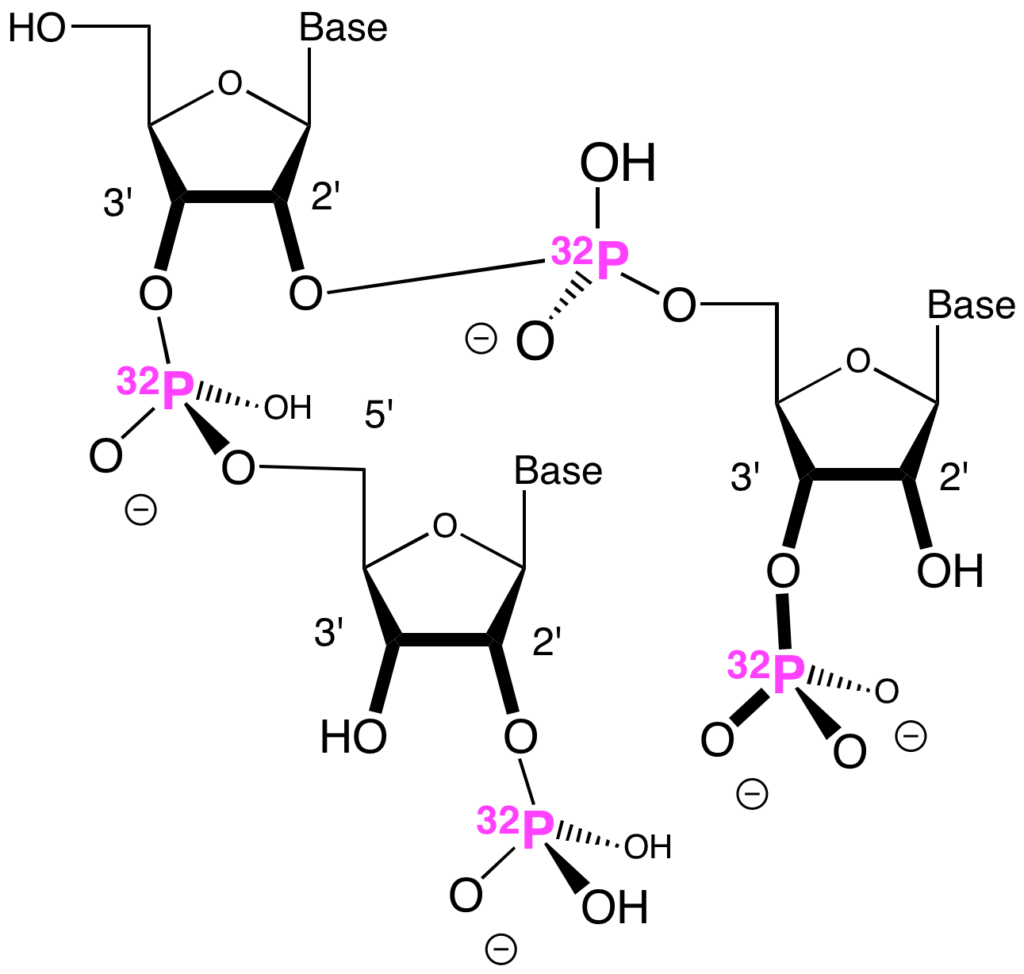
In an electric field, RNA moves through a gel at a rate that slows as the RNA gets longer. In initial experiments, we used this electrophoretic mobility to estimate the range of lengths of polyribonucleic acid molecules formed on basalt. From this mobility, we estimated the range of lengths of the polyribonucleic acids formed on basalt. That range was “100 to 200 nucleotides”, the number that I gave above.
Now, in an electric field, branched RNA moves through a gel a bit more slowly than linear RNA made from the same number of nucleotides. Thus, if we had used electrophoretic mobility alone to estimate the range of lengths of polyribonucleic acid formed on basalt, and if we had assumed that it was linear, we would have overestimated its length if the polyribonucleic acid (in fact) contained branches. Even if the branched units were too few to be noticed among the degradation products produced by alkaline digestion.
We actually had addressed Jack’s concern by asking: How serious might this overestimate be? The literature provides a guide.
For example, Coppin and Silverman [3] compared the electrophoretic mobility of a linear 34mer RNA molecule with the electrophoretic mobility of a branched 34mer RNA molecule. In the branched RNA molecule, 25 nucleotides were in the linear part, and 9 more were in the branch.
The branched 34-mer moves as if it were a linear 39-mer. That is, electrophoretic mobility would say that the branched RNA molecule has 15% more nucleotide building blocks than it actually has.
Now, in our first experiments, we used electrophoretic mobility to estimate that the length of the polyribonucleic acid made on basalt was 100-200 nucleotides, assuming it to be linear. But what if it contained a single branch? Let us apply a 15% adjustment. Now the length range is 85 to 170 nucleotides.
Still impressive.
Two branches, perhaps 80-160 nucleotides long.
Still impressive.
In fact, there is no way that a highly branched polyribonucleotide with, let us say, 50 nucleotides can move as a linear polyribonucleotide that has 100-200 nucleotides. Here, highly branched DNA structures [4] are better studied than RNA structures, but the conclusion is inescapable. Basaltic glass assembles a lot of nucleoside triphosphates.
However, since we always prefer “science” over “hype”, we sought a way to independently estimate the length of the basalt-synthesized polyribonucleic acid. Here, we used a different tool, a tool known as “ultrafiltration”.
An ultra-filter is a membrane with holes punched through it. Different membranes have different sized holes. These are metricked using globular proteins. Of course, polyribonucleic acids behaves differently from proteins as it encounters the hole. This means that we must map the size of the hole in different ultra-filters to their ability to pass RNA having different sizes. Again, literature was our guide.
For example, Manzano et al. [5] calibrated ultra-filters in their work using ultrafiltration to control the quality of RNA molecules used in therapeutics. They measured the passage of RNA molecules 70-247 nucleotides long through membranes with holes rated at 50000, 100000, and 300000 daltons. This range was comparable to the range of polyribonucleic acid synthesized on basalt. For example, they found that a 247 nucleotide RNA molecule was >90% retained at a defined time by a 100000 dalton membrane.
We ourselves used ultrafilters to estimate the size of the polyribonucleic acid that basaltic glass had made. In one experiment, ultra-filters with 100000 dalton holes retained about 25% of the basalt-synthesized polyribonucleic acid. This requires that most of the polyribonucleic acid have lengths greater than at least ~120 nucleotides.
Of course, this number is also a range, and branching might influence the calibration. But as with electrophoresis, branching cannot misrepresent a molecule that is in fact 50 nucleotides long into one that appears, by ultrafiltration, to be a molecule 100-200 nucleotides long. And even if the polyribonucleic acid made on basalt had a distribution of lengths down to 50 nucleotides, it is still impressive.
Ultrafiltration experiments answered objections raised by other critics. For example, some peer reviewers advised the editors that the product whose structure we had assigned as polyribonucleic acid was not covalently linked at all, but rather was a non-covalent aggregate.
Yes, this criticism ignored the experiments that recovered nucleoside 2′- and 3′-monophosphates, including radioactive materials. All discussed above. But in the culture of journal editing, the reviewers never carry a burden of proof. Editors will (nearly always) credit a criticism made by a referee, even if it contradicts facts. Such criticisms must be addressed.
Therefore, we dug out some more basaltic glass, made some more polyribonucleic acid, and repeated the ultrafiltration experiments. But this time, we ultrafiltered the polyribonucleic in the presence of acid. And then in the presence of base. And then in the presence of organic solvents.
Each time, the polyribonucleic acid held together as only covalent bonds would allow.
Those not trained in chemistry also raised fanciful ideas. For example, some critics suggested that the material might contain phosphate linkages between the 5-oxygen of one nucleotide and the 5′-oxygen of another. Again, these could not be formed if our understanding of chemistry is correct.
But we dug out some more basaltic glass, made more polyribonucleic acid, and did more analyses. For example, we treated the polyribonucleic acid made on basalt with an enzyme (ribonuclease) that degrades natural RNA. The degradation went smoothly, consistent with the structure assigned already.
Here, we made assumptions about how ribonuclease worked. We thought to ourselves: “If the ribonuclease cleaves only 3′,5′-linkages and leaves 2′,5′-linkages untouched, then we might be able to quantitate the ratio of the two linkages in the polyribonucleic acid, by quantitating the amount of nucleoside monophosphates that we recover relative to the amount of undegraded linkages.”
By this metric, it appears as if most of the linkages are 3′ -> 5′. But that required that we understand how bonds are transformed by the enzyme ribonuclease. How do we know that ribonuclease is absolutely specific, cleaving only 3′,5′-linkages? Elisa found literature suggesting that the linkage specificity of ribonuclease need not be absolute.
We thought of reverse transcribing the polyribonucleic acid to get DNA. But here, we would not necessarily be able to interpret the results, given unknowns relating to the rearrangement of bonds effected by the enzymes. Could we be certain that the enzyme did not read through branches? How would it interact with various linkages in various numbers and various orders?
All good questions worth answering.
But as new experiments were done to address increasingly complex challenges from referees, we began to encounter what we might call “sociological problems”.
We were running out of glass.
We had obtained authentic rock glasses of various types from the United States Geological Survey, a few grams each of reference material. As we did experiment after experiment required by referee after referee, our stash got smaller and smaller.
We contacted the USGS to get more rock glasses. We were told that the USGS was no longer providing them. COVID. Or something.
And, of course, we had long run out of money for “origins” research. Several requests to the Simons Foundation had been declined. An application to NASA failed. As Tom Wolfe said in The Right Stuff, “No bucks, no Buck Rogers”.
So sociology brought the “science” to a (temporary) end. At this point, there was no alternative but to publish exactly what our structural analysis had showed:
Triphosphates on basaltic glass give polyribonucleic acid having a range of lengths of 100-200 nucleotides, judging both by electrophoresis and ultrafiltration. The fractions of 2′,5′ and 3′,5′-linkages are unknown but, to the extent that ribonuclease digests only the latter, 3′,5′-linkages are abundant. There may be some branching, but this does not materially change these conclusions.
Of course, by publishing, we were able to raise funds to return to “origins” work. Interestingly, our combination of precise models for Hadean geological environments and rigorous organic chemistry attracted a joint venture of the NSF geology and the NSF molecular biology directorates.
And the shortage of glass? My next post will describe my solution to this problem, which involved my traveling Iceland, whose surface is largely basaltic glass. Now we can do Dan Brown studies and enzyme studies and all of the other studies that anyone might want. We can improve the structural model for the ribonucleic acid made on basalt. We can quantitate branching and linkage types. And we can distribute kits to high-schoolers.
As I was wrapping this essay, it occurred to me that these events offer the opportunity to make another comment on culture, primarily to those training to become scientists.
Public presentation of results will be an important part of your jobs. Yes, publication is advertisement, or “hype”. Indeed, the US government a few decades ago demanded that journal articles be marked “advertisement”, if the authors paid journal publication fees.
As this story illustrates, the timing of publication is easily driven by sociological factors. When will the students graduate? What samples are available? Where can we get funds to do the research.
In their different cultures, different fields have different tolerances.
In prebiotic chemistry, that tolerance is high. For example, some suggest that joining geology to chemistry is a “new modus operandi” [6] for research to understand life’s origins.
In a discipline where so little is known, perhaps tolerance of such “hype” should be high.
Some more reading:
[1] Wolfe-Simon, F., Blum, J. S., Kulp, T. R., Gordon, G. W., Hoeft, S. E., Pett-Ridge, J., … Oremland, R. S. (2011) A bacterium that can grow by using arsenic instead of phosphorus. Science, 332(6034), 1163-1166.
[2] Kaku, M. (2010) Life as we don’t know it. NASA’s discovery of an ‘‘exotic’’ DNA changes everything. Wall Street Journal, December 6, 2010.
[3] Coppins, R. L., Silverman, S. K. (2004) A DNA enzyme that mimics the first step of RNA splicing. Nature Structural & Molecular Biology, 11(3), 270-274.
[4] Li, Y., Tseng, Y. D., Kwon, S. Y., d’Espaux, L., Bunch, J. S., McEuen, P. L., Luo, D. (2004) Controlled assembly of dendrimer-like DNA. Nature Materials, 3(1), 38-42.
[5] Manzano, I., Vezeau, G., Salis, H., Zydney, A. L. (2020) RNA size and 3-dimensional structure determine ultrafiltration behavior of small RNA molecules. Separation and Purification Technology, 237, 116372.
[6] Powner, M. W., Sutherland, J. D. (2011) Prebiotic chemistry: A new modus operandi. Philosophical Transactions of the Royal Society B: Biological Sciences, 366(1580), 2870-2877.
[7] Benner, S. A., Bains, W., Seager, S. (2013) Models and standards of proof in cross-disciplinary science: The case of arsenic DNA. Astrobiology 13, 510-513.
Hi Steve,
A very neat and succinct response to the criticism from your old intellectual sparring partner Jack Szostak. The fact that your ‘proof of concept’ used the old classical structure analyses tools first employed by the late, great Dan Brown (to decipher the molecular structure of RNA) as well as modern ultrafiltration techniques, is indeed very elegant, almost 70 years on from the work of Dan and Watson and Crick. Let me add two references to Dan Brown’s 1953 work:
Brown D. M., Fried M. and Todd A. R., The Determination of nucleotide sequence in polyribonucleotides. Chem and Ind. (1953), 352-353.
Brown D. M., Todd A. R. et al Structure of adenylic acids a and b. Nature (1953), 1184-1186.
Thank you for these references, Kevin. I am now part of the dwindling bridge to this past, having had the pleasure of meeting and dining with Dan Brown before he passed away.
Cheers Steve, likewise I am also very much a part of the same dwindling bridge, having had Colin Reese, (to whom Dan Brown was a friend and mentor, in Alexander Todd’s group in Cambridge in the mid-1950s) as my PhD supervisor. He really enjoyed reading your latest prebiotic RNA syntheses and nucleoside triphosphate formation papers!
FYI: the discussed paper from Elisa and her team is an open access article and hence available to everyone, so you can make your own judgment about the process and diligence.
Dear Steve,
I like the way you handled that. You can surely be a thoughtful thinker.
I enjoy your writing style: knowing Jack Szostak before he was “Jack Szostak”. And “‘culture’ is what you think when you are not thinking.”
I concur with the cultural problem, graduate student tenures, covid, obtaining more samples, and sinusoidal funding. You and I are more aligned than some might think because we have the same cultural upbringing: synthetic organic chemistry. I just wish you had really watched my videos on OOL before commenting on them. You probably would have then gone with the science rather than the hype.
God bless,
Jim Tour
Some years ago I was involved in some work with Martin Brasier on the possible roles for glassy, vesicular volcanic rock in the origin of life: https://www.liebertpub.com/doi/abs/10.1089/ast.2010.0546
We gave four motivations for thinking about this:
(1) During eruption it develops the highest surface-area-to-volume ratio known for any rock type.
(2) It is the only known rock type that floats as rafts at the air-water interface and then becomes beached in the tidal zone for long periods of time.
(3) It is exposed to an unusually wide variety of conditions, including dehydration.
(4) It has a remarkable ability to adsorb metals, organics, and phosphates as well as to host organic catalysts such as zeolites and titanium oxides.
We focused on pumice but should really have talked more about scoria!
Thanks for your work moving this whole area forwards.
Sean